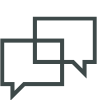
Stories
Steven Prescott: Alleviating Chronic Pain—From Garden Snails to Allodynia
It was in high school that Steven Prescott first held a brain in his hands. It belonged to a fetal pig that he was dissecting in a biology course, and he remembers staring at it and marveling at how the human brain, though it functions similarly to a pig brain, is capable of so much more. As he continued his education, his fascination with the inner workings of the central nervous system only deepened.
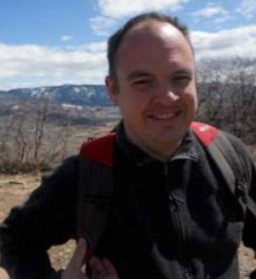
“It seemed to me that the brain really controls your reality, in the sense that everything you perceive ultimately gets filtered through your brain, and whether you’re perceiving something correctly, or incorrectly has to do with how the brain is functioning,” Prescott says. “So, with devastating neurological conditions or mental-health problems—schizophrenia, things like this—brain disorders change everything.”
Prescott’s fascination with the brain remained following this experience, and he grew more curious about the brain’s capabilities. After completing high school, Prescott continued his studies at McGill University, where he completed his first research experiments in neuroscience. He studied the tentacle-withdrawal reflex of the garden snail, Helix aspersa,an invertebrate model organism for learning and memory research. Prescott’s project was to record the electrical activity of the snail’s neurons while observing habituation, or sensitization, of the tentacle-withdrawal reflex . While repeatedly touching the tip of the tentacle, he looked for changes in neuronal activity that underlie changes in the reflex.
“In this particular reflex, there’s one giant neuron called C3 that plays a particularly important role,” Prescott says. “A bunch of upstream sensory neurons funnel into this one major motor neuron. One of the things that came out of those experiments was finding that you had the simultaneous development of different learning processes. The mechanisms mediating sensitization and habituation were happening in different parts of the circuit. What that means is that they were ultimately competing with one another in order to dictate how the circuit, how the reflex, was changing.”
This early experience would come to shape how Prescott thought about his research strategy and his interest in the study of pain. From these experiments, he learned the pitfalls of having a reductionist approach to understanding complex biology. “You have to be careful about studying the parts of the system in isolation, because many of the interesting things are based on how these different parts interact with one another,” Prescott says. He began to experiment with the idea of approaching neuroscience research from a systems biology perspective, with a focus on nonlinear dynamics—a mathematical approach for understanding complex relationships and how they develop.
“Studying snail reflexes was a great learning experience, but it wasn’t may passion. Chronic pain seemed like a research question that could keep me busy for a long time. And it was also an important clinical problem that fit with my longstanding high school aspirations of becoming a neurologist, or a neurosurgeon, or something like that.”
Around the same time that Prescott was experimenting on garden snails and learning nonlinear dynamics, he was introduced to the field of chronic pain in a class taught by McGill Professor Ronald Melzack. Melzack is known for having described the gate control theory of pain, which explains how pain is more than simple cause and effect—it is influenced by other neural inputs and stimuli in the nervous system.
“Melzack was a very impassioned lecturer, and he convinced me that chronic pain is not only a huge clinical problem, but also a really interesting neurobiological problem,” Prescott says. “This fit well with the sort of research I was doing. Studying snail reflexes was a great learning experience, but it wasn’t my passion. Chronic pain seemed like a research question that could keep me busy for a long time. And it was also an important clinical problem that fit with my longstanding high school aspirations of becoming a neurologist, or a neurosurgeon, or something like that. So that’s what led me to the M.D./Ph.D. program at McGill.”
For his graduate research, Prescott completed studies in electrophysiology, recording the electrical activity of neurons in a region of the spinal cord called the superficial dorsal horn. The neurons within this region are important for pain processing, and it is at the stage when sensory information from the periphery reaches the superficial dorsal horn that the gate control theory applies.
“With the gate control theory, there’s a lot of processing that happens at the first synaptic relay point that controls what sensory information is relayed up to the brain, and I think there’s also a lot of room there for that information to get corrupted. This is to say, I think of chronic pain as a neural coding problem. So, the question becomes, what is the normal neural code, and how is this going wrong?” explains Prescott.
Upon graduating from McGill, rather than continue with a clinical residency, Prescott joined the lab of Terry Sejnowski at the Salk Institute as a postdoctoral fellow. There he gained new skills in computational mathematics that he could apply to the field of neuroscience. He spent his time in the Sejnowski lab running simulations, learning mathematical models, and mastering the foundations of computational techniques.
In 2008, Prescott was hired by the University of Pittsburgh, where he set up his own lab as an assistant professor, and in 2009 he was selected for the Rita Allen Foundation Award in Pain as part of the inaugural cohort.
“I think it was the first major external award I received,” Prescott recalls. “When I was just getting started, it was helpful to get that first one under my belt. That early success helps, I think, with the future success. I can say that getting that early award was helpful in getting the data that helped get the later NIH awards and so on and so forth. Leveraging that early success allowed me to do the research that I had wanted to do—getting back into the spinal cord and trying to look at how the circuit was organized and how that organization could be changed or how the function could be changed under pathological conditions that were associated with chronic pain.”
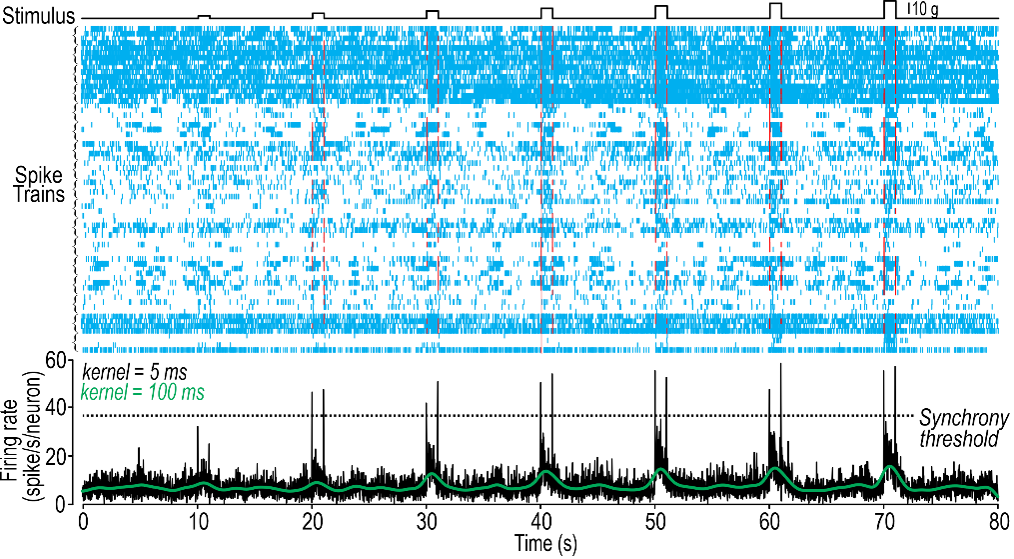
Today, Prescott is a professor at the University of Toronto and a senior scientist at the Hospital for Sick Children. The Prescott lab explores how neurons and neural networks process information, including information related to pain, and how pain can be perceived incorrectly in conditions like allodynia. Prescott and his team have observed that the answers to these questions lie in how neurons transmit information through action potentials.
Here, Prescott describes his research, how he uses computational mathematics to understand chronic pain, how emerging technology is changing his research methods, and what his life is like outside of the lab.
Can you speak about the research you are conducting at the Hospital for Sick Children and the University of Toronto?
Neurons use short pulses of electricity called action potentials to communicate information. But neurons can become altered in how they generate those action potentials—they can become hyper-excitable, which is to say they generate too many action potentials. So, we’re really trying to understand which neurons become hyper-excitable and how they become hyper-excitable. This ultimately depends on ion channels, which are the little pores inside the neuron membrane that allow ions—the charged particles—in and out of the cell. Ion channels control the generation of these action potentials, but it’s not any one ion channel that’s responsible for a neuron becoming hyper-excitable. In fact, we know that there are dozens of different types of channels in any one neuron, and many of those ion channels can become dysregulated. How is it that the interactions between these ion channels are altered in such a way as to cause the neuron to become hyper-excitable?
Getting back to this idea of nonlinear dynamics, we ask, how are the emergent properties of the neuron changed by what might seem like subtle changes in the ion channels? These changes conspire to cause qualitative, dramatic changes in how the neuron behaves, and how neurons interact at the circuit level to control what the circuit is doing. Altogether this changes how you perceive sensory stimulation. We’re trying to understand how those pathological changes develop and how drugs can be used to normalize some of these ion channel changes and restore normal sensory processing.
How are some of the new tools and emerging technology affecting your science, or some of the emerging science that you’re seeing? Is it changing any of your approaches to how you’re doing your work?
I think increasingly as technology has changed, it is easier to collect the data, but then the challenge shifts because you have so much data. The challenge becomes trying to interpret that data, to grapple with just the sheer amount of data, and to take full advantage of it. It’s not like you necessarily have more of the same data, but you can actually now start asking additional questions. I’ll give one example: A lot of my research involves trying to record the electrical activity of neurons. We can do that with electrodes, but now we have multi-electrode arrays that can allow us to record many neurons simultaneously. Or we can do this with GCaMP imaging—a genetically encoded calcium sensor—so that we can image the activity of many neurons simultaneously. Not only does that give us more information about what each neuron is doing, but it also allows us to ask additional questions about how the neurons are cooperating at a network level. This is important for neural coding—to ask not only what is the rate of activity of each neuron, but are there any patterns, correlations, between the activity of those neurons.
What do you do when you’re outside the lab?
I like having uninterrupted time to read in areas of science that I’m not so familiar with, because I find that it’s really exciting to connect up disparate ideas. I’m also interested in the history and philosophy of science. How do we know things? Is our thinking biased by prevailing theories? Are we asking the right questions? I think it’s helpful to be introspective about how we do science.
I spend my free time with my wife and daughters. All of us do karate together a couple times per week. And all of us share a passion for the outdoors. Whenever given the chance, on a long weekend or a summer vacation, we get out of the city. The more remote, the better. We especially love multi-day hikes into the backcountry. No cell reception. Very peaceful.